Research & Projects
On this page
- Structure, function, and modulation of protein-protein interactions and protein networks
- Tethering and targeting cysteine reactivity
- Probe and drug discovery using high-content imaging
Structure, function, and modulation of protein-protein interactions and protein networks
Our lab has been interested in how proteins recognize each other and how we can use this information to develop inhibitors of protein-protein interactions. Recently, we have taken on the broader challenge of understanding how proteins interact in networks to modulate arrays of cellular activities. Inhibiting or stabilizing one interaction might have global effects on these interacting networks. Our long-term aim is to develop context-specific modulators of protein-protein interactions to interrogate biology and develop innovative new medicines.
Allosteric and protein-protein interaction modulators of p97
The AAA+ ATPase p97/VCP is a master regulator of proteostasis and is involved in functions as diverse as chromatin remodeling, ER-mediated degradation, and golgi remodeling. p97 forms a homohexamer with a four-domain architecture: an N-terminal domain that functions as an anchor site for cofactor/adaptor/substrate recognition, two ATPase domains D1 and D2, and a C-terminal region that also binds adaptor proteins. The D1 and D2 domains form two stacked rings, creating a central pore through which unfolded protein strands can pass. p97 is reported to interact with up to forty regulatory enzymes and adaptor proteins. Each directs p97 to a specific protein quality control pathway. Due to p97’s important role in maintaining proteostasis, mutations are implicated in cancer, neurodegenerative disease, and the degenerative disorder multisystem proteinopathy (MSP1, also called IBMPFD and VCP disease). Using small molecules and protein engineering, we aim to understand p97’s cellular activities by developing function-selective modulators of p97.
p97 is involved in diverse cellular activities.
Structural studies have revealed at least three distinct conformations of p97 which can depend on the identity of bound nucleotides, bound proteins, and the presence of disease-causing mutations at the ND1 interface. These conformations, as visualized by cryo-EM, are shown in the movie below. This movie iterates among the three major, symmetrical conformations observed in the static cryo-EM reconstructions. ATPase-dependent structural changes provide insight into how p97 mediates unthreading of ubiquitinated substrates through its central pore. In addition, ATP induced perturbation of the N-domain suggests a mechanism for the engagement/disengagement of p97 associated proteins in changing cellular contexts (2.3 Å resolution cryo-EM structure of human p97 and mechanism of allosteric inhibition, Science, 2016; p97 Disease Mutations Modulate Nucleotide-Induced Conformation to Alter Protein-Protein Interactions, ACS Chem Biol, 2016).
We have employed biochemical and biophysical techniques to understand the interplay between nucleotide-binding, cofactor-recognition, p97 conformational change, and age-related cellular changes such as pH in the function of p97. These outcomes will provide insights into small molecule drug discovery for this protein target.
Current Work
We are exploring multiple approaches to identify compounds that bind to multiple allosteric and protein-interacting sites on p97. Our current efforts use multiple approaches to lock the N-D1 domains into the ATP-like and ADP-like conformations. These tools will be used to evaluate the importance of conformation in p97’s biological functions. Protein engineering tools like phage display are discovering protein-based antagonists of specific p97/adaptor proteins. We are also engineering the enzyme itself to characterize how p97 responds to nucleotide-binding, cofactor-recognition, and age-related cellular changes.
Stabilizing protein-protein interactions with 14-3-3 proteins
To understand and manipulate PPI networks, we want to be able to systematically inhibit or stabilize one interaction at a time. PPI stabilizers are relatively uncommon, and dedicated screening approaches to identify PPI stabilizers are virtually nonexistent.
In collaboration with Ottmann Lab and Brunsveld Lab (Eindhoven University of Technology), we use site-directed disulfide tethering to identify compounds that stabilize 14-3-3/client interactions. We then crystalize and optimize these compounds to stabilize the specific 14-3-3/client interaction. The figure below shows two examples of stabilizing disulfide compounds; these compounds can be discovered through tethering to 14-3-3 (left) or to the client protein (right). These compounds are then tested for their effects on 14-3-3/client protein function in cells to better understand the client protein’s role in disease.
Expanding on these projects, we are systematically evaluating the breadth of the disulfide trapping technology for discovering 14-3-3/client stabilizers. Future projects will apply this approach to new classes of PPI. We also use biophysical and cellular approaches to understand the thermodynamic and kinetic parameters that govern 14-3-3/client biology. These data will us to predict the outcome of stabilizing the PPI and assess the expected biological response.
See also:
- Fluorescence Anisotropy-Based Tethering for Discovery of Protein-Protein Interaction Stabilizers, Sijbesma, et al, ACS Chem Biol, 2020
- Site-Directed Fragment-Based Screening for the Discovery of Protein-Protein Interaction Stabilizers, Sijbesma et al, J Am Chem Soc, 2019
Tethering and targeting cysteine reactivity
Disulfide trapping is a fragment screening method that allows the targeting of specific sites of interest on proteins. In this approach, a protein containing a native or introduced cysteine residue near a site of interest is screened against a library of thiol-containing, drug-like fragments under conditions that promote thiolate-disulfide exchange. Fragments that form both noncovalent and covalent binding interactions near a cysteine residue are stabilized and therefore persist, whereas fragments that bind non-specifically are reduced away.
The fragment-modified protein is stable and is readily detected by high-throughput mass spectrometry, allowing identification of the bound fragment. By screening pools of distinct-mass fragments, thousands of fragments can be screened per week. The low hit rate observed (about one to five percent) speaks to the selectivity of the method and permits the screening of proteins containing multiple thiols without multiple fragment binding. Crystallographic studies have demonstrated that disulfide-trapped fragments bind in the same mode as the corresponding free (untethered) fragment, thus validating this approach for the design of noncovalently bound ligands.
In the figure above, multiple potential pathways exist to optimize candidate fragments for potency, selectivity, and cell-based activity. The noncovalent portion of the fragment can be optimized through structure-guided design and/or empirical SAR. Using an “extended tethering” approach, the first fragment is modified to include a new thiol that can serve as new “bait” to screen for a second fragment that binds in a nearby site; converting the resulting disulfide bond leads to a high-affinity ligand (top). If the coordinating cysteine residue is present in the wild-type protein, the disulfide can also be converted to a weak electrophile (center); we are exploring this approach for non-catalytic cysteine residues near substrate-binding sites. Finally, if a second fragment/ligand is known to bind nearby, the two fragments can be linked to form a high-affinity, noncovalent compound for further medicinal chemistry (bottom).
See also:
- Tethering
- A Liquid Chromatography/Mass Spectrometry Method for Screening Disulfide Tethering Fragments, Hallenbeck, et al, SLAS Discovery, 2017
- Targeting Non-Catalytic Cysteine Residues Through Structure-Guided Drug Discovery, Hallenbeck, et al, Curr Topics in Med Chem, 2017
- Covalent targeting of acquired cysteines in cancer, Visscher, et al, Curr Opin Chem Biol, 2016
Novel inhibitors of caspase-6
Caspases play key roles in inflammation, apoptosis, and neurodegeneration. However, it has been very difficult to identify cell-active, selective caspase inhibitors. Caspases-2 and -6 are particularly implicated in neuronal development, axonal pruning, and excitotoxicity. Caspase-6 is also associated with cleavage of tau protein in Alzheimer’s disease (AD); tau cleaved by Caspase-6 has been found in neurofibrillary tangles and in brains with mild through severe forms of AD, and elevated levels of active Caspase-6 has been found in AD brains.
To address the role of caspase-6 in neurodegenerative disease, we set out to develop highly selective and potent cell-active probes of caspase-6. In collaboration with the Renslo Lab and Genentech, we identified potent noncovalent inhibitors of caspase-6 that exhibit exquisite selectivity over other caspases by binding uncompetitively to the enzyme/substrate complex (Mechanistic and Structural Understanding of Uncompetitive Inhibitors of Caspase-6, Heise, et al, PLoS One, 2012).
Our current work is focused on probes that bind covalently to caspase-6. Using our disulfide-trapping technology, we identified covalent inhibitors that bind to a non-conserved cysteine residue in the caspase-6 substrate-binding groove. Working with the Renslo Lab, we have optimized electrophilic analogs that show >1000-fold selectivity for binding/inhibiting caspase-6 over other caspases. We solved co-crystal structures of compounds bound to caspase-6 and are using structure to design more potent inhibitors. Current work is focused on demonstrating cellular activity and selectivity. If successful, we will use these compounds to address the fate of tau and neurons with active and inhibited caspase-6.
Probe and drug discovery using high-content imaging
High-content analysis (HCA) is a powerful assay methodology that combines high-throughput microscopy and automated image analysis. We hypothesize that cell-based screens designed with primary cells will more faithfully translate to model organisms and humans than do screens designed in cell-culture lines. Towards this aim, we have developed HCA for screening whole parasitic organisms (Giardia, T. cruzi, Leishmania, S. mansoni), developed in partnership with the Center for Discovery and Innovation in Parasitic Diseases (CDIPD).
ATOM
Currently, drug discovery is a slow, costly, and sequential process associated with low rates of success. ATOM is a collaboration between academia, industry, and government institutions with the mission to accelerate drug discovery by integrating systems modeling, machine learning, and human-relevant complex in vitro models. The Arkin Lab at UCSF provides the experimental data that is needed to optimize preclinical safety predictions. With drug-induced liver injury (DILI) as one of the leading causes of attrition during drug development, we focus on building a computational platform that can accurately measure and predict DILI at the organismal and population levels.
The ATOM Consortium’s platform of accelerating the current drug discovery process and its founding members.
We have developed a suite of high-content cell health assays that measure hepatocyte health using structural and functional biomarkers of hepatotoxicity. Biomarkers include cell count, nuclear area, reactive oxygen species (ROS), mitochondrial membrane potential and permeability, cellular ATP, and glutathione (GSH) levels (Multiparametric High-Content Assays to Measure Cell Health and Oxidative Damage as a Model for Drug-Induced Liver Injury, Curr Protoc in Chem Biol, 2020). Additionally, bile acid transporter inhibition is also investigated in collaboration with the Giacomini Lab. Together, we have screened hundreds of compounds with known animal and/or human liver data. These mechanistic and multi-parametric data coupled with Quantitative Systems Toxicology (QST) tools and machine learning (ML) models will enable us to predict DILI potential based on the structure of a proposed drug lead and contribute to transform drug discovery into a more rapid, integrated, and patient-centric model.
Current high-content imaging workflow. Acquired multiplex images then undergo nuclear or cellular segmentation to define the areas of interest and allow normalization of data per cell. Features are then extracted according to the rationale of each particular readout. Features include, but are not limited to, cell count, signal intensity, and area of a particular object. Numerical data from extracted features are then plotted in graphs. Created with BioRender.com and adapted from Pohan et al., 2020.
Live imaging of Schistosoma
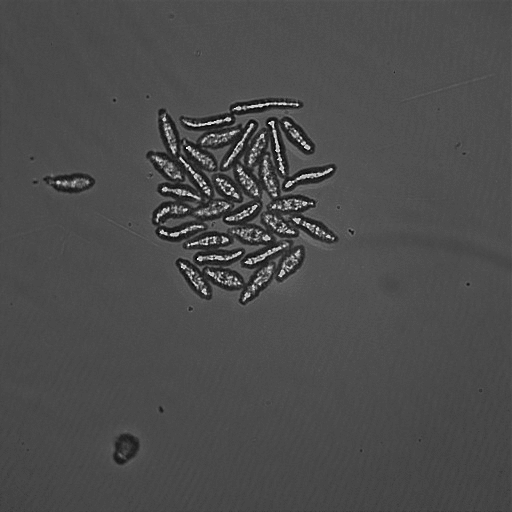
Schistosomula, the youth-stage of the human parasite S. mansoni, swimming to the center of a well in a 96-well plate.
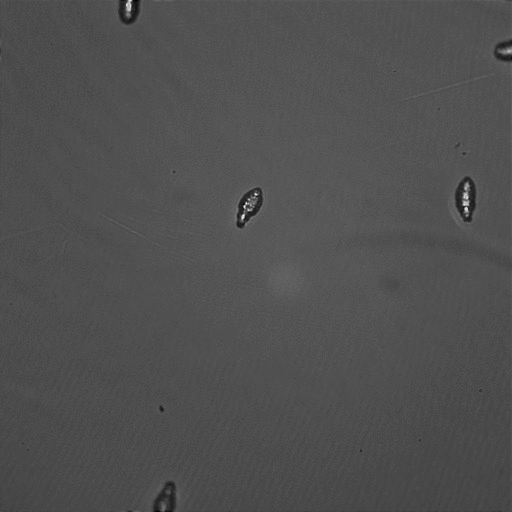
Schistosomula, the youth-stage of the human parasite S. mansoni, swimming to the center of a well in a 96-well plate.
Collaborating with Conor Caffrey, PhD (UCSD) and Rahul Singh, PhD (SFSU), we are studying the causative agent of schistosomiasis. Schistosomes are parasitic flatworms that infect 3.5% of the world’s population yet have been recalcitrant to modern methods of drug discovery. We have developed an automated high-content screening platform to quantify chemically-induced, multi-parametric responses of living Schistosoma mansoni. We demonstrated the importance of measuring motility in defining drug phenotypes/mechanisms and provided unprecedented time- and dose-quantitation. Data can be queried using SchistoView. We aim to develop drug screening for helminths as a fully quantitative science and to provide tools to explore parasite development and drug mechanism of action (A multi-dimensional, time-lapse, high content screening platform applied to schistosomiasis drug discovery, Communications Biology, 2020).
High-content imaging tools provide the opportunity to study high-resolution biology in a high-throughput and quantitative format. Using complex cell culture and whole organisms, we aim to solve biological problems and also to advance high-content imaging technology.